SEVENTEEN
Meanwhile, at Via Panisperna
While Ettore languished in the throes of creative failure, bereavement, a bad stomach, and an unused cock (whether for lack of a he or a she), life went on at Via Panisperna. Belatedly, Fermi had seen Ettore’s wisdom, duly pushing on to become the number-one expert in the field of neutrons and nuclear forces. And yet the Majorana curse was upon him: “Physics is on the wrong road” was Ettore’s pronouncement when Amaldi force-fed him the happenings taking place at Via Panisperna. These events would become Fermi’s terminal trauma, worse than his early scoop by Pauli. They concerned something of paramount historical importance: the fission of uranium. And the dark secret stored in the basement of Via Panisperna.
The incidents surrounding the discovery of the fission of uranium—the process behind the atomic bomb—could be used as a definition of fuck up. To understand how such a blunder could have happened at Via Panisperna in 1934, one must backtrack a few months and note that Fermi was then riding on a huge high. In such circumstances, people relax and lower their guard. The fuck-up can then detonate in their faces, unexpectedly and unchallenged.
The time was the academic year 1933-1934—just after Ettore retreated into his shell—and it could be called Fermi’s annus mirabilis, in analogy with Einstein’s 1905 and Newton’s 1666. He’d been severely stuck in his own prejudices up until then, and apart from Ettore, so had everyone else at Via Panisperna. As late as 1932, evidence in Fermi’s notebooks indicates that he didn’t believe in the neutron. Fermi had been marooned: Perusing his notes is an exercise in humility. How could someone who’d be so crucial to a scientific revolution be so far off at first?
In October 1933, the seventh Solvay Conference took place, ostensibly to resolve the mystery of the nucleus. Part of a series set up by Belgium industrialist Ernest Solvay, these exclusive invitation-only meetings gathered the top minds in physics.46 Fermi was invited. Ettore wasn’t. But the congress was dominated by Ettore’s theory of strong forces, as reported by Heisenberg. What Ettore perceived as his 1932 failure—his unfinished symphony—passed unnoticed. This congress could have been a moment of triumph for Ettore, but by this time he was no longer in public circulation.
One wonders just what Fermi’s feelings toward Ettore’s success were, but in any case they had the right effect: They spurred him on to shake off his obstinacy regarding the neutron’s existence and to shift from atomic to nuclear physics. This unblocked his creativity and led to his best scientific period.
Fermi first set to work on his godson, the neutrino. In December 1933 he produced a landmark paper on beta decay, developing the tools still in use today for computing the probability of a beta transmutation, with the emission of an electron and a neutrino. To gravity and electromagnetism, Ettore and Heisenberg had added the strong force, capable of binding the nucleus together; Fermi now postulated a fourth force—the weak force—responsible for beta decay and the creation of neutrinos. With his theory he predicted a plethora of probabilities for “weak” processes: decay rates of isotopes, the inverse beta-decay process (later used to detect the neutrino), and others. The central constant in “weak theory” is nowadays called Fermi’s constant.
“A fantastic paper . . . a monument to Fermi’s intuition,” was the judgment of a major nuclear physicist decades later. And Fermi was very proud of his accomplishment, as he enthusiastically explained it to the Boys congregated in the Alps for a spell of skiing that winter. “I could hardly sit . . . bruised as I was from several falls on icy snow,” related Segrè. Needless to say, Fermi’s paper, “Tentative Theory of Beta Rays,” was rejected by the magazine Nature as “too remote from physical reality.” This irritated Fermi a great deal.
Next, Fermi steered the whole research at Via Panisperna to experimental nuclear physics, much to the consternation of Rasetti, who felt more at home with atomic physics. Overnight, Fermi converted from a “neutron denier” into one of its greatest authorities. Ernest Rutherford, the leading nuclear experimentalist, wrote to him, “I congratulate you on your successful escape from the sphere of theoretical physics! You seem to have struck a good line to start with. You may be interested to hear that Professor Dirac also is doing some experiments. This seems to be a good augury for the future of theoretical physics.” Thus Fermi embarked on the route that would lead him to uranium—and his biggest blunder.
In January 1934, the couple Irène and Frédéric Joliot-Curie accidentally discovered artificial radioactivity. As I explained earlier, isotopes existing in trace amounts in nature are unstable and decay into other elements with the emission of radioactive radiations: alpha, beta, and gamma rays. But by bombarding stable (i.e., nonradioactive) elements with alpha particles (obtained from naturally radioactive isotopes) the Joliot-Curies produced new, man-made radioactive isotopes. This was a dazzling discovery—yet another installment in the alchemical dream of transmutation. Yet a bad omen hung over the discoverers at once. “We are entitled to think that scientists, building up or shattering elements at will, will be able to bring about transmutations of an explosive type,” said Frédéric Joliot-Curie in his Nobel Prize address. He went on to express his fear of a cataclysm should these transmutations “spread to all the elements of our planet”—perhaps the first recorded statement of nuclear Armageddon.
After reading the Joliot-Curies’ paper, Fermi threw himself into the production of artificial radioactivity. The Joliot-Curies had used alpha particles as their bullets (which, recall, are made up of two neutrons and two protons); Fermi opted for neutrons instead. He was ridiculed by the experts (Otto Frisch labeled his experiments as “really silly”) because the neutron sources available at the time were much, much weaker. But Fermi realized that neutrons were vastly more efficient in producing transmutations. Alpha particles are positively charged, and so are all nuclei; therefore, there is an electric repulsion between the two, forming a barrier to be overcome before the alpha particle can penetrate the nucleus and cause a transmutation. No such barrier afflicts the neutron, because it is electrically neutral. Neutron sources were weaker, but their bullets much more effective. So Fermi gambled on neutrons.
The trouble was that the Via Panisperna group had no experience whatsoever in experimental nuclear physics. To make matters worse, Rasetti, Fermi’s main hand with experiments, was undergoing a crisis of scientific belief (the first of many.) On a whim, Rasetti had recently abandoned his experiments, to go on an extended holiday to Morocco “in pursuit of an elusive phantom, the hope of finding something, somewhere that would give him satisfaction and a sense of fulfillment,” according to Laura Fermi.47 Undeterred, Fermi jumped alone into the dark.
It is at this point that I have to reveal the nature of the treasure locked in the basement of Via Panisperna. It was inside a safe, and the key was in the pocket of Professor Trabacchi. For medical purposes only, inside the safe lay one gram of radium, that important radioactive element first isolated in large amounts by Madame Curie. It was worth about 700,000 lire. By comparison, the annual equipment budget at Fermi’s disposal was 40,000 lire, already ten times the normal budget of other physics departments. It was out of the question for Fermi to buy this radium.
But radium gives off a gas called radon. Professor Trabacchi—reinforcing his nickname of the Divine Providence—happily allowed Fermi to pump the gas from the safe into his lab. With some clever adaptation, Fermi converted this radon into a good source of neutrons.48 Fermi began bombarding elements with neutrons and by March 20, 1934, had achieved artificial radioactivity in aluminum. An urgent telegram was sent to Morocco, summoning Rasetti back to Rome. The Via Panisperna Institute had joined the nuclear race.
The Boys began a systematic investigation of the production of artificial radioactivity using neutrons. By midsummer, they’d produced no fewer than three-dozen new “artificially made” radioactive isotopes. But in the middle of so much mindless sweat, a drama occurred, requiring serious brain power. Accidentally, they hit on one of their most important discoveries, an essential tool in nuclear physics to this day.
One day, Segrè and Amaldi were performing an irradiation, obediently doing as they were told by Fermi, who was about to depart for a conference in London. They obtained the required result—a given transmutation that led to a gamma-ray emission—which Fermi duly communicated during his conference. But the next week, Amaldi repeated the experiment, just to make sure, and was embarrassed to find a totally different result. Upon being told about the discrepancy, Fermi assumed human error and was incandescent. As Segrè put it, “On his return to Rome he scolded us for our apparent carelessness. We were not only unhappy, but confused, because we could find no fault with the various experiments that gave contradictory results.”49 Amaldi and Segrè were deeply hurt at having their ears boxed by the Pope, but even worse, Fermi—apparently not trusting them—set newcomer Bruno Pontecorvo on the case.
When Pontecorvo examined the problem, the mystery only deepened: He confirmed that the same target, when bombarded with neutrons, sometimes led to one type of transmutation, other times to another. He also observed the same phenomenon in other elements, not only in the case found by Segrè and Amaldi. Furthermore this was not the kind of randomness they’d come to expect from quantum mechanics, a randomness ruled by well-established odds and leading to repeatable experiments. This was total chaos without any pattern or rule. On Monday one result would be obtained, on Friday another. The outcome depended on which corner of the lab, by whom, and on which table the experiment was performed. Even quantum mechanics wasn’t this crazy. It looked as if there were ghosts at Via Panisperna.
For months they puzzled, seeking a control, a “placebo,” some sort of reproducibility in their experiments. They went through the dreadful game of perhaps this, perhaps that, perhaps something else altogether was changing every time they did their experiments. At some point in the cruel charade, they wondered whether their makeshift neutron source failed to keep a constant “power.” To test their hypothesis, they encased the source in lead, in the hope of slowing down the neutrons and obtaining a pattern of changes in their irradiations. Slower alpha particles are less effective in producing artificial radioactivity; perhaps a similar effect was present with neutrons. But lead had no effect whatsoever on their experiments. Whereas something else—and, infuriatingly, they didn’t know what—clearly did.
It was then that Fermi had a brainwave. One morning, during the examination season, when they were all very busy with chores, he chose on a whim to filter the neutrons with a block of paraffin instead of lead. No reason whatsoever, just a “why not?” To everyone’s surprise, the production of artificial elements markedly increased ! But this was deeply counterintuitive to them: With alpha particles, any filtering reduced their speed and therefore their ability to induce radioactive transmutations. Not so with neutrons, evidently. Why? Fermi went home for lunch plus a siesta.
When he came back, he had the explanation: They were being utterly stupid. Of course faster was better for alpha particles, because they had to overcome the repulsion from the nucleus. But neutrons felt no such electric barrier; and the slower they were, the longer they spent inside the nucleus, and so the higher their chance of causing a transmutation. (This is the sort of lateral thinking that would have caused no problem to Ettore—I note—but at least Fermi got there eventually.)
Fermi then realized that what was slowing down the neutrons was the hydrogen contained in the paraffin, and that some desktops in the lab were made of marble, others of wood, their hydrogen content thus being quite different. “Fantastic! Incredible! Black magic!” Fermi reportedly howled. In one stroke, two discoveries had been made: that hydrogen is the “moderator” of choice to brake neutrons; and that the slower the neutrons were, the more effective they were in producing nuclear reactions. The ghost at Via Panisperna had been exorcised—the experiments now made sense. And thus the Boys discovered slow neutrons, an absolutely essential tool in nuclear physics today, used in everything from weapons to nuclear-power stations.
What followed describes well the Boys’ hormonal state at the time. Leaping from the building, screaming and shouting, they went to test their new toy with hydrogen-rich water, using the largest volume of that fluid at hand: the fish pond in the courtyard (see Figure 17.1). Rasetti raised salamanders there, but now, without a second thought, they jumped in, submersing the target and neutron source to repeat the experiment underwater. The caretaker came out, protesting:
“What the hell are you doing?”
“Experiments,” was the reply.
The pond of the Via Panisperna Institute.
“But why here in the fountain? You’ll scare the fish, maybe they’ll die.”
But unaware that they were now probably condemned to die of cancer, the fish swam on unperturbed; it was the men who yelled and frolicked in the water who seemed to be on the verge of heart attacks. Their experiment had worked: Water slowed down the neutrons even further, increasing manifold their ability to cause transmutations.
Later, they went to Amaldi’s, whose wife had a typewriter, to compose a paper reporting their new discovery. Segrè recalled, “Fermi dictated, while I wrote; Rasetti, Amaldi and Pontecorvo paced the room excitedly all making comments at the same time.” According to Laura Fermi, “They shouted their suggestions so loudly, they argued so heatedly about what to say and how to say it, they paced the floor in such audible agitation . . . that the Amaldis’ maid enquired timidly whether the guests had all been drunk.”
Next morning, Corbino, more levelheaded, foreseeing the industrial applications of the discovery, suggested they patent it. “I can’t forget,” recalled Pontecorvo, “the sincere, childish and vocal laughter with which the suggestion of Corbino was received, who, seeing Fermi and the others laugh so much, observed brusquely ‘You are young, you don’t understand anything.’”
And they really didn’t. The only one who might have foreseen the implications of what had just been discovered was Ettore. But he had retired from the world.

During the maelstrom that was the Via Panisperna lab in that period, it was at some point decided to systematically bombard every element in the periodic table with neutrons, starting with hydrogen and helium and proceeding onward in an orderly manner. Tasks were distributed: Fermi did the calculations, organized the experiments, and led their execution; Amaldi prepared the “electronics” and the measuring devices; Segré was invested with the errand of procuring all the elements in the periodic table: hydrogen, helium, lithium, beryllium, boron, carbon, nitrogen, oxygen, etc. In some cases, it was trivial to track down a given element; in others, it was a laborious job. Eventually, Segrè unearthed a theology student who collected elements as a hobby (it takes all sorts to make this world). As Laura Fermi described it, “When in going down his list Emilio reached cesium and rubidium (two soft, silvery metals seldom used in chemistry), Mr. Troccoli [the theology student] got them down from the highest and dustiest shelf, saying: ‘You can have these free.’”
The repetitive work began. To avoid contamination, elements would be irradiated by the neutron source at one end of a corridor and then tested for artificial radioactivity at the other end. If short-lived isotopes were produced, this involved a swift run down the corridor, and Amaldi and Fermi prided themselves on being the fastest runners. A Spanish professor, seeking to meet “His Excellency Prof. Fermi” was thus surprised to be nearly knocked down by Fermi, his lab coat flying out behind him, as he approached the sound barrier. “The Spanish visitor could not conceal the depth of his thwarted expectations,” according to Laura Fermi. One also wonders what Professor Antonino Lo Surdo thought of these athletics.
In general, neutrons cause light elements to become lighter, say, by emitting an alpha particle (their A decreasing by three and their Z by two; see Figure 17.1). This is possible because their nuclear charge is not too high (their Z—or number of protons—being low) so the electric barrier for the emission of a positively charged particle is not overpowering. But for heavier elements, with their larger Z and so larger nuclear charge, this is not possible, so that when they are hit by neutrons, still heavier elements are produced. The archetype involves the neutron being captured by the nucleus, leading to an isotope with the same number of protons but an extra neutron; this then undergoes beta decay; i.e., a neutron inside the nucleus converts into a proton with the emission of an electron and a neutrino. So bombarding an element with Z and A eventually produces another with Z + 1 and A + 1 (see Figure 17.2).50 It all made sense and was consistent with the nuclear theory worked out by Ettore and Heisenberg. And so they proceeded, element by element, checking the predicted patterns, bored and slightly braindead. Until they finally arrived at uranium.
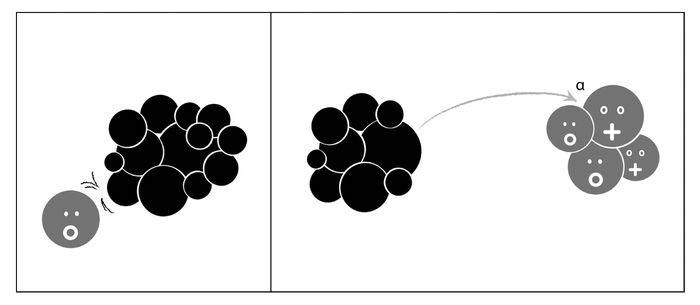
Figure 17.1: Neutrons running into light nuclei render them even lighter by stimulating the emission of an alpha particle. In the process, the nucleus gains a neutron but loses an alpha particle: Therefore its A decreases by three and its Z decreases by two.
Figure 17.2: When bombarding heavy nuclei with neutrons, a different process is preferred. The neutron is first absorbed, leading to a surplus of neutrons over protons in the nucleus. The intermediate isotope thus created then undergoes beta decay: One of its neutrons converts into a proton with the emission of an electron and a neutrino. So bombarding with neutrons an element with numbers Z and A eventually produces another with both Z and A increased by one.
Element number 92—uranium—is the last natural element of the periodic table (i.e., the one with the largest number of protons.) Nothing with a higher Z exists in nature, and it was thus expected that by bombarding uranium with neutrons, element 93 and possibly 94 would be produced.51 The political potential of such a discovery didn’t escape Senator Corbino. If it all followed the usual molds, a new element or elements would be produced, not merely radioactive isotopes of existing elements. Someone would have to christen the new entries in the periodic table: Just think of the PR opportunity!
Shortly after the first irradiation of uranium with neutrons, Senator Corbino gave a speech to the Academy of the Lincei, with the king and the press in attendance. Displaying his brilliant oratory skills, he announced the discovery of the new element(s). Even though he acknowledged Fermi’s “prudent circumspection,” he stated that as far as he was concerned, the “production of this new element is already securely established.” This set the press braying as usual. Add the reigning fascist regime and you shouldn’t be overly surprised by the headlines: “Fascist Victories in the Field of Culture” mooed one paper; “In the Fascist Atmosphere Italy Has Resumed Her Ancient Role of Teacher and Vanguard in All Fields” cackled another. One paper went as far as to report that “Fermi present[ed] a small vial of the element 93 to the Queen.” It was suggested that the new element be christened Mussolinium—but someone pointed out that, with the new element being so short-lived, this might be seen as an insult by the Duce. In the end the choice fell on Ausonium and Hesperium, derivative names for Italy, and thus suitably nationalistic.
The circus triggered by Corbino annoyed Fermi immensely: “I have seldom seen him in such a dark mood as after the speech made by Corbino” said Segrè later. First because Fermi didn’t like publicity, then because he was very methodical and wanted to make sure nothing had been overlooked. He spent several sleepless nights while Oscar D’Agostino, a chemist recently lent to him by the Divine Providence, performed the relevant checks. Weeks passed by, with Fermi scared to death that a major mistake might have been relayed to the press. Then, as Fermi’s notebooks reveal, he, too, felt sure they’d produced elements 93 and 94. As late as 1938, in his Nobel Prize speech, Fermi talks about this “discovery.”

The “fuck-up” was complete. Later, Fermi would be bitterly aware that, in spite of all his achievements, he’d missed the greatest discovery of all. Before a picture commemorating him at Chicago University, Fermi once said, “It represents a scientist who was unable to discover fission.” He also later admitted, “We didn’t have enough imagination.” For someone so traumatized by being scooped (and what it meant in the shadow of his dead brother), it must have been maddening. It’s not as if he didn’t have time to catch up with his error: It took almost five years for the issue to be resolved. And yet the writing was on the wall.
It’s no accident that uranium is the last element of the periodic table to occur naturally. Everything with a higher Z is extraordinarily unstable. So when a neutron is captured by uranium, nature prefers to do something dramatically different: The uranium nucleus breaks into two much smaller nuclei (krypton with Z = 36, and barium with Z = 56) releasing a large amount of energy. Crucially, further neutrons are released during this “fission” process, capable of restarting the process in an infamous loop called a chain reaction. The rest is history: The atomic bomb, nuclear power stations . . . the nuclear age, in summary, is epitomized by fission. First done at Via Panisperna: except that no one noticed.
It would be easy to blame D’Agostino—responsible for the chemical tests—but that wouldn’t be fair. D’Agostino’s tests were standard issue and were carried out scrupulously. But the problem is that in examining the decay products of an irradiation, the chemist wasn’t allowed to ask the general question, “What are they?” Instead he could only ask specifically, “Is it this; is it that?” And since no one knew the chemical properties of element 93, all he could do was to rule out that what had been produced were known elements. He checked the vicinity of uranium, for example elements 91 (protactinium), 90 (thorium), 89 (actinium), 88 (radium), and many others. He proved that these hadn’t been produced; and thus the argument in favor of element 93 strengthened. He didn’t check for elements 36 (krypton) or 56 (barium): Why the hell would anyone bother with that? It would contradict anything known in nuclear theory.
Well, in fact, someone did realize their fallacy straight away: Chemist Ida Noddack—by all accounts a Nazi and an unbearable person. In a paper published in a chemistry journal, she showed that the outcome of bombarding uranium could well be two pieces of comparable size, much larger than an alpha particle, but much smaller than “Mussolinium.” She was ignored by Fermi, to whom she sent her paper. Segrè would later admit, “The possibility of the fission of Uranium escaped us even though it was signaled to us by Ida Noddack. . . . The reason for our skepticism is not clear to me even today.”
Noddack was also ignored by all the other nuclear physicists. Fission simply wasn’t a physical possibility according to the understanding of the nucleus encapsulated in Ettore and Heisenberg’s theory of the nucleus. Ida Noddack discovered fission blindly where the others failed to see it because they had eyes. Ettore may have been the one exception. He was so disparaging of his own work—so aware of its limitations—that he may well have seen that the theoretical arguments were flawed . . . and the possibilities this opened with regard to uranium.
But Ettore was a recluse by this point, even though Amaldi kept him informed of these developments. It wasn’t until 1939 that Hahn, Meitner, and Frisch set the matter of uranium fission straight. Just think of the historical implications, had Ettore brought fission into the world—with all its applications—as early as 1934. It’s not surprising that conspiracy theories explain his disappearance by having him kidnapped by secret-service agents.