ELEVEN
Creation and Annihilation
Sometimes I think that despite their scientific differences, Ettore and Dirac would have been the best of friends. They found themselves at opposite ends of a scientific dispute, yet their mathematical minds worked in similar ways, and their personalities had much in common. But to the best of my knowledge they never met. What a pity: It would have made for some first-class anecdotes.
Dirac was Ettore without his gregarious side, to the point of borderline if not outright autism. He seldom spoke, but when he did the outcome was minimalistic, logically crafted insanity. He was incapable of not thinking literally. At a conference someone got up and said, “Professor Dirac, I don’t understand how you derived the formula on the top right-hand corner of the blackboard.” To this he responded with silence. When the chair inquired why he didn’t answer the question he replied, “That was not a question, it was a statement.”
Perhaps Dirac’s genius derived precisely from the savant streak present in some autistics. When a Russian colleague gifted him Dostoyevsky’s Crime and Punishment his opinion was “It’s nice, but in one of the Chapters the author made a mistake. He describes the Sun rising twice on the same day.” Was this uncanny ability to discern irrelevant detail an autistic-savant trait? It certainly led him to new mathematics where others had failed. But on the very few occasions when he commented on his own work, he claimed to be guided by “mathematical beauty,” a concept he considered superior even to simplicity. “It is more important to have beauty in one’s equations than to have them fit experiment”—the ultimate victory of Platonism.
Dirac was even more taciturn than Ettore, and his inability to make small talk was legendary (that his wife, Manci, never shut up was apparently unrelated). A visitor to Cambridge narrated, “The weather outside was very bad, and since in England it’s always respectable to start a conversation with the weather I said to Dirac, ‘It’s very windy, Professor.’ He said nothing at all, and a few seconds later got up and left. I was mortified, as I thought that I had somehow offended him. He went to the door, opened it, looked out, came back, sat down and said, ‘Yes.’”35
Unfortunately, I have the dreadful feeling that none of this outlandish behavior is actually funny; that it was involuntary, something he couldn’t help, and caused him much suffering. Dirac was severely abused as a child. If Ettore was traumatized by his mother, Dirac endured an authoritarian and sadistic father. “I never knew love or affection when I was a child,” he’d say cryptically in his old age. His brother committed suicide while still very young, and Dirac blamed his father. When Dirac won the Nobel Prize in 1933, he invited only his mother to his lecture. The extent of the abuse to which he was subjected has never been fully investigated. For someone so quiet and private, it’s harrowing to hear that Dirac once publicly said that his father was the only person he ever loathed.

Paul Dirac was born in Bristol, England, on August 8, 1902, and, like Ettore, first trained as an engineer. His aversion to experimental work, however, soon led him to switch to mathematics. While Ettore was attracted to Via Panisperna, Dirac fled to Cambridge, that ivory tower of lunacy, where his behavior passed largely unnoticed. In the early 1990s, when I was a fellow at what had been Dirac’s college, he was still fondly remembered (unlike Manci, who terrified generations of misogynist academics). At the high table, “the Dirac” was still in use as a unit of “verbal output,” similar in nature to the carat or the microgram for weight.
In 1926 Dirac finished his Cambridge University PhD degree, with a thesis titled “Quantum Mechanics,” the equivalent of titling a book Poetry or Science Fiction. But it’s a befitting title: It’s where the foundations were laid down for what had been a fragmentary hotchpotch. Overnight, the twenty-five-year-old Dirac became the leading authority in quantum theory. Dirac’s formulation of quantum mechanics led to all the modern offshoots, including attempts to quantize gravity.
After completing his PhD, Dirac embarked on an even more ambitious project: “relativistic quantum mechanics.” In its initial formulation, quantum mechanics was only applicable to particles moving much slower than the speed of light. Einstein’s theory of relativity gives a radically different picture of the universe when things move fast. Clocks tick more slowly, distances shrink, objects become “heavier” and more difficult to accelerate. A relativistic quantum theory that unified quantum mechanics and special relativity was lacking.
No one knew how to describe quantum phenomena for particles moving close to or at the speed of light. Dirac set about filling the gap. The result was Dirac’s theory of the electron. And most infamously, the antiworld.

Dirac’s discovery was made purely via mathematics, and at first it didn’t conform to experiment at all. Had it not been for his obsession with “mathematical beauty” (to the detriment of experiments if need be), I suspect that Dirac would have given up and the antiworld been found only much later. He worked with formulae and abstract concepts, conciliating Einstein’s theory of special relativity and quantum mechanics in his mind, setting up a mathematics embodying both. It took him several months of trial and error in 1927 to hatch his theory. But when he finally devised an equation describing a quantum-mechanical particle (with spin and all the quantum trimmings) moving close to the speed of light, he got a surprise. His equation—nowadays called Dirac’s equation—described not one particle, but two.
Somehow the mathematics doubled the number of particles in the theory once quantum mechanics and special relativity were married. Taking the electron as an example, Dirac’s equation contained solutions with the correct mass, spin, and
Figure 11.1: The positive energy “bowl.” At zero speed, the electron has a rest energy E = mc2, associated with its mass at rest, m. As the speed increases (for both positive and negative speeds), its energy increases. (The bowl extends upward forever and is actually infinite.)
charge and, crucially, with positive energies (see Figure 11.1). But there was also a whole world of identical electrons, with the same mass and spin but negative energies (see Figure 11.2).
Physicists don’t like negative energies and for good reason. If you accelerate a negative-energy particle you gain rather than spend energy. Sounds like a cool solution to the world’s energy crisis, but things just don’t work that way. Furthermore, I’m not sure we’d like the free ride: We wouldn’t be able to stop the process, and it would end in apocalypse. In the same way that all matter rolls to its minimal-energy state, electrons with negative energies would indulge in a runaway behavior, where they would accelerate more and more (causing their energy to become more and more negative), meanwhile flooding their environment with positive energy, in a spiraling, uncontrollable mess.
The solution to the problem found by Dirac is ingenious. Nowadays it’s considered heuristic, and there are more mathematically rigorous alternatives, but it’s still an intuitive depiction.36 Dirac cured the instabilities associated with these negative energies with the following recipe: Fill all the negative-energy states with particles, so that no state is left free. And call that “nothing,” or the vacuum (see Figure 11.3).
Figure 11.2: Dirac’s inverted energy bowl, servicing negative-energy electrons. The rest energy of such electrons is negative, and their energy decreases (it becomes more negative) as their speed increases. It therefore costs nothing (indeed energy is gained) to accelerate a negative-energy electron. A runaway instability follows.
Figure 11.3: Dirac’s cure to the negative-energy instability: Fill all the negative energy states with particles so that no state is left free and define such a “sea of electrons” as “nothing.” Negative-energy electrons now cannot run away down the bowl, because the bowl is entirely full.
If you accept this “redefinition” of the vacuum, stability is accomplished. Negative energy particles can’t run away into even more negative states (shedding an overdose of positive energy into the rest of the world), because all such states are already filled. Neither can positive-energy particles jump into negative-energy states. The universe becomes stable because the negative-energy states are all filled by default. Crucial to Dirac’s argument is the realization that you can’t put two particles into the same state (at least not for fermions, precisely according to the principle Fermi failed to grasp ahead of Pauli).
But if this “Dirac sea” of negative-energy particles is declared to be the vacuum, then what is “something” that exists, “something” that isn’t part of “nothingness”? One only measures differences between things, so reality—existence—should be what’s new with respect to Dirac’s sea. Such as an electron with positive energy—a regular electron, negatively charged as usual (see Figure 11.4). Or a hole in the Dirac sea: the failure of one of the negative-energy states to be filled by an electron. Such a hole would be perceived as a deficiency in negative electric charge and in negative energy. It would therefore be seen as a particle with positive energy and positive charge (see Figure 11.5 and Figure 11.6). Less debt is equivalent to more money: Likewise, less negative energy and charge is equivalent to more positive energy and charge. Thus, the prediction of the antielectron, or positron: a particle with the same mass and spin as the electron, but opposite electrical charge—and with positive energy, of course. The antiworld was unveiled.
I’m told that on cruises, if the ship’s engines are turned off in the middle of the night, everyone wakes up startled as if by the loudest of dins. The engine’s hum has become the vacuum. Its absence is now perceived as a loud, sleep interrupting, antinoise. A similar argument willed the positron into existence.
In fact, the electron is not alone and any charged particle, by the same token, must have a corresponding antiparticle with the same mass and spin but opposite charge. There is an antiproton, whose experimental discovery earned Segrè the Nobel Prize in 1959. The antiproton has a negative charge like an electron but the same mass as a proton. Likewise for all charged particles.*
Figure 11.4: A positive-energy electron hovering above Dirac’s negative-energy sea. The sea passes unnoticed (because it’s “nothing”) and only the positive-energy electron is noted.
Figure 11.5: A hole in Dirac’s sea would necessarily be seen as “something,” since it constitutes a blemish in the vacuum, or the nothingness.
Our world is primarily made of matter, and antimatter is rare, produced only in physicists’ accelerators or by cosmic rays. Just as well. Because something dramatic happens when matter meets antimatter: They annihilate.
Consider Dirac’s picture of the positron as a hole in a sea of electrons filling all the negative-energy states. If a regular electron (i.e., an electron with positive energy) meets such a hole it happily jumps into it (things love going downhill). In the process it sheds the large energy difference between the two states in the form of light (typically two photons). At the end we have the hole filled and no positive-energy electron; i.e., we have what we defined as nothing. The electron and positron have annihilated, releasing their inner energy in the form of very powerful light (see Figure 11.7). The amount of energy released in these annihilations is enormous. Just plug the electron mass into E = mc2, and you’ll find that you wouldn’t want to be anywhere nearby. A bomb made of matter and antimatter brought together would be more than a thousand times more powerful than an atomic bomb with the same mass. Except that I’m not sure how such a bomb could be manipulated without detonating immediately.
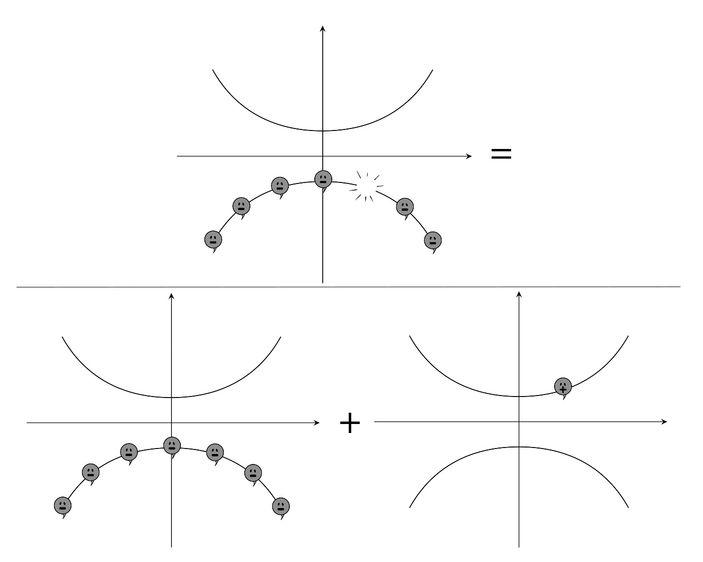
Figure 11.6: A hole in Dirac’s sea is equivalent to the sum of a perfectly filled sea and a particle with opposite qualities to that missing in the hole; i.e., a particle with positive energy and charge (notice the “mouth” of the positron). Thus was the positron predicted.
Conversely, a large amount of energy (say, in the form of two photons) could make an electron in the negative-energy sea jump into a positive-energy state (see Figure 11.8). At the end, with respect to the vacuum, we have a positive-energy electron plus a negative-energy hole; i.e., an electron plus a positron. Two very energetic particles of light can therefore create a pair of matter and antimatter particles in a process reciprocal to their annihilation. Creation and annihilation are two sides of the same coin—and are the most distinctive hallmark of Dirac’s theory.

Nowadays Dirac’s theory is lauded as one the greatest achievements of the twentieth century, but no one believed it at first (and Pauli took the piss out of it* For neutral particles the story is more involved. For example, the photon doesn’t have an antiphoton, or seen in another way, the photon and the antiphoton are identical. But the neutron does have a very distinct antineutron. As for the neutrino . . .
tremendously). In his first paper, Dirac wrongly stated that the antielectron was the proton; Oppenheimer duly pointed out that particles and their corresponding antiparticles must have the same mass. The proton, recall, is 1,836 times “heavier” than the electron.
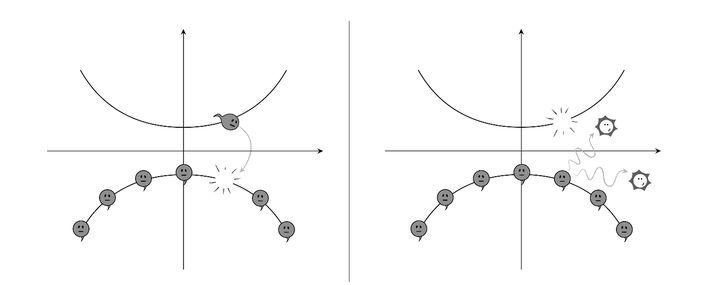
Figure 11.7: An electron-positron annihilation. If a positive-energy electron meets a hole in Dirac’s sea (a positron) it may jump into it, releasing the energy difference in the form of two very energetic particles of light. At the end, the hole is filled at the expense of the positive-energy electron. We have, therefore, a perfect Dirac sea, or a vacuum: The two particles have annihilated.
Figure 11.8: The creation of an electron-positron pair. In the converse process to annihilation, two very energetic photons knock a negative-energy electron into the positive band. With respect to the vacuum, we end up with a positive-energy electron plus a negative-energy hole; i.e., an electron plus a positron.
But Dirac found the mathematics in his theory beautiful, so he stuck to his guns. OK , so his theory didn’t describe the electron and the proton: It described the electron and another particle with the same mass and spin but opposite charge, which had yet to be seen. Somebody would eventually find the positron, he was sure. Dirac thus started the trend of postulating new, as yet unobserved particles that has continued to this day, when everyone fancies themselves a little Dirac. Thousands of unobserved particles fill the tomes of theoretical physics, waiting to be detected in order to provide the proposer with a suitable Nobel Prize. Too good to be true, their enthusiastic creators seemingly fail to notice.
Of course, the story with Dirac was different, but as Ettore came into the picture there was no evidence for the positron. Ettore wasn’t amused. To him it was all utter rubbish: negative energies, particles that didn’t exist, and whatnot. His anti-Dirac reaction would lead him to the Majorana neutrino, but Ettore’s claim to Nobelization is much more profound: His neutrino is merely the bastard child of much deeper work. What really worried Ettore was what he perceived as the sheer nonsense of Dirac’s sea and its negative energies. This opposition to Dirac’s theory led him to a masterpiece, “Relativistic Theory of Elementary Particles with Arbitrary Spin” published in 1932: his unfinished symphony.
But then, in the months that followed its publication, a strange set of events was set in motion in Ettore’s life.